Specification
Temperature | He bath cryostat: 4.2 K - 300 K (2.5 K with pumping) |
Magnetic fields | Oxford IPS 120: up to 6.5 Tesla (Reversible) |
Pressure |
Easylab Pcell: up to 30 kbar |
Rotation | Uniaxial 360° |
Optimum measurement value | 10-9 - 106 Ωcm |
General Description
Even though it sounds classics, resistivity measurements have always been and still are a powerful technique to study the nature of materials. Introduced by the German physicist Georg Ohm in early 1800s, this method has been vastly developed since then. The idea itself is simple: one applies current and measures voltage or vice versa. In our lab, a multi-purpose resistivity measurement setup has been developed utilizing a He cryostat (Figure 1). With this setup, we have been able to investigate the electronic properties of many exotic materials, ranging from Mott-insulators to topological semimetals. Our setup allows us to go down to 4.2 K and, with pumping, to 2.5 K. Additionally, magnetic field of up to 6.5 T can be applied. There are two sample holders available, one of them for magnetoresistance, the other one for high-pressure measurements. With this setup we can measure resistivity from 10-9 to 10 6 Ωcm with high precision.
DC Magnetoresistance and High-Pressure Measurements Configuration
Depending on the sample, 2 or 4 contact measurement techniques can be utilized. In total, there are 8 pins that can be used (Figure 2). The sample holder itself can be used to mount samples from 5 mm down to 100 micron in size. Since we have 3 nanovoltmeters, 3 measurements can be done simultaneously. The sample holder itself is 360° rotatable; with this, measurement error due to the alignment can be minimized. We can arrange the setup for the measurements of longitudinal and transversal magnetoresistance, as well as for Hall measurements.
With this setup, we are able to reveal important properties of the samples studied including, magnetoresistance (Figure 4), charge concentration and mobility (Figure 5), gap opening (Figure 6) and Hall resistance (Figure 7).
References:
[1] M. B. Schilling, A. Löhle, D. Neubauer, C. Shekhar, C. Felser, M. Dressel, and A. V. Pronin, Phys. Rev. B 95, 155201 (2017).
[2] D. Neubauer, PhD thesis, Stuttgart University (2017).
[3] A. Löhle, E. Rose, S. Singh, R. Beyer, E. Tafra, T. Ivek, E. I. Zhilyaeva, R. N. Lyubovskaya and M. Dressel, J. Phys.: Condens. Matter 29, 055601 (2017).
Contact
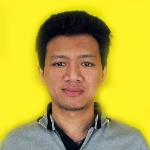
Lucky Maulana
Dr.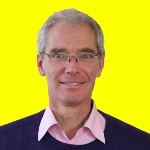
Martin Dressel
Prof. Dr. rer. nat.Head of Institute